BSM-SG Eternity: Quantum Architecture Comparision
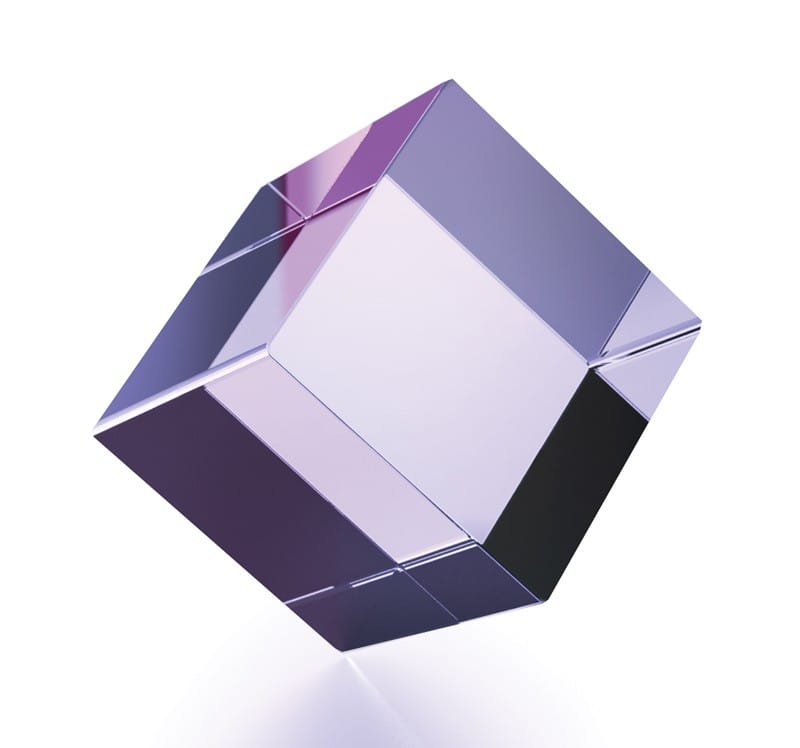
BSM-SG Eternity is a solid-state quantum system using trivalent ytterbium (Yb³⁺) as a quantum information carrier. Qubits are implemented via the spin states of Yb³⁺ ions, implanted or doped into a crystal lattice (solid-state matrix). Their states are controlled through microwave transitions between hyperfine levels, with the system being magnetically stabilized—applying a static magnetic field that separates the energy levels and ensures a so-called "clock" transition, less sensitive to noise.
For initialization and manipulation, optical excitation may also be used, but the main method of control is via microwave pulses, similar to nuclear magnetic resonance. Qubit readout is optical—via laser excitation of the Yb³⁺ ion and detection of emitted fluorescence. The spin state influences the photoluminescence intensity (optically detected magnetic resonance, ODMR), allowing the bit to be read with a photodetector (e.g., photodiode).
Architecturally, BSM-SG resembles systems with spin defects: the quantum carriers are fixed in a solid material (no vacuum traps), enabling integration with classical electronics (e.g., embedded photodiodes for readout). The platform is expected to be compatible with universal quantum algorithms since it uses discrete qubits and logical operations, allowing programming via frameworks like Qiskit. As of now, it is not publicly known whether the architecture is patented, but the unique combination—Yb³⁺ in solid state + microwave control + optical readout—is likely the subject of patent filings by its developers.
AWS Ocelot (Cat Qubits – Superconducting Photonic Resonators)
AWS's Ocelot is an experimental quantum processor that uses so-called "cat" qubits. In this system, quantum information is stored in coherent states of microwave photons within superconducting resonators—each cat qubit is implemented in an oscillator circuit (a superconducting microwave loop resonator) that can exist in two widely separated amplitude states, analogous to Schrödinger's cat.
These resonator-based qubits are integrated on a chip alongside additional superconducting circuits. Control is achieved via microwave signals applied to the resonators and auxiliary transmon qubits—for instance, two-photon pumps and stabilizing circuits maintain the oscillators in their "cat-like" superposition states. By their nature, cat states suppress bit-flip errors, focusing quantum operations primarily on correcting phase errors.
Measurement in Ocelot is electronic—using superconducting circuit-based sensors. Each resonator qubit is coupled with an auxiliary quantum element (e.g., another qubit/oscillator), serving as a detector for phase fluctuations. The state is read via microwave dispersive measurement, similar to standard transmon systems.
Architecturally, Ocelot is a planar superconducting chip featuring 5 cat resonator qubits, supplemented by 4 additional qubits for error detection, all fabricated using semiconductor technologies. The chip operates at deep cryogenic temperatures (around 10 mK) to maintain superconductivity.
Although Ocelot is not publicly accessible, software compatibility is theoretically feasible—since it realizes logical qubits, it can execute quantum circuits analogous to those in Qiskit, provided the hardware is integrated through a suitable software interface. AWS already offers the Braket platform for accessing quantum devices. Patents: Ocelot introduces a novel form of hardware quantum error correction, so it is likely that AWS and Caltech have filed patents covering the combination of cat qubits and scalable integration.
The technology, however, is quite distinct from BSM-SG—instead of atomic spins, it uses photons in superconducting resonators, and the readout is not optical. Therefore, there is no direct overlap with BSM-SG.
---
Ion Traps (IonQ, Quantinuum)
Trapped ions are a well-established quantum technology, represented by companies such as IonQ and Quantinuum (Honeywell). These systems use individual atomic ions (e.g., $^{171}$Yb⁺ at IonQ, or $^{171}$Yb⁺ and $^{138}$Ba⁺ at Quantinuum) as quantum carriers. The ions are held in ultra-high vacuum electrode traps—e.g., a linear Paul trap—where they form a crystal-like chain.
Qubit control is typically laser-based: each ion has internal energy levels (e.g., hyperfine spin states) that are manipulated via precisely directed laser beams. At IonQ, each Yb⁺ qubit is addressed by an individual laser beam (via an acousto-optic deflector), allowing for two-photon Raman transitions equivalent to spin rotations. Two-qubit operations are implemented via the ions’ collective oscillations—i.e., interaction through shared vibrational modes (Molmer-Sorensen gates), induced by bichromatic laser pulses. (Quantinuum employs a similar approach, using additional ion species for cooling and other enhancements.)
Some ion-based architectures, such as Oxford Ionics, explore microwave control instead of lasers, using RF and gradient fields—but industry standards still favor laser precision. Qubit readout is performed via state-dependent fluorescence: after the algorithm runs, each ion is illuminated with a "readout" laser. Depending on its internal state (|0⟩ or |1⟩—usually F=0 or F=1 hyperfine levels), the ion either fluoresces brightly or remains dark. Specialized detectors (e.g., photomultiplier tubes, EMCCD cameras, or superconducting photon counters) capture individual photons from each ion, delivering highly reliable output (>99% fidelity).
These systems have modular architectures: trap on an optical table, multiple lasers for cooling, control, and readout (e.g., 369 nm for readout, ~355 nm for gate transitions, 935 nm for repumping, etc.). IonQ and Quantinuum achieve long coherence times through the use of clock transitions—for example, $|F=0, m_F=0⟩$ and $|F=1, m_F=0⟩$ in $^{171}$Yb⁺, which are first-order insensitive to magnetic fields. They still apply a weak magnetic field (~5 Gauss) to define the quantization axis while minimizing decoherence.
This magnetic stabilization resembles that in BSM-SG, which also likely uses clock transitions of Yb³⁺ with minimal B-field sensitivity. Software compatibility: Ion-based quantum computers are universal and already available via cloud platforms. IonQ, for example, is accessible through Amazon Braket, Microsoft Azure, and supports Qiskit integration. Quantinuum has its own software stack (tket, Quantinuum API) but supports standard quantum circuits. Thus, users can program ion-based machines using high-level languages.
Patents: Numerous patents exist in this domain—IonQ has patented techniques for integrating optical elements with chip traps, and Honeywell/Quantinuum has developed mechanisms for combining trap modules, among others. While standard methods (laser cooling, fluorescence readout) are scientifically known, engineering implementations are protected.
Comparison with BSM-SG: Both use Yb ions and optical readout, a clear similarity. The difference is that BSM-SG fixes Yb³⁺ in a crystal matrix, while IonQ works with Yb⁺ in vacuum—allowing full laser access versus solid-state integration. BSM-SG relies more on microwaves for addressing due to the challenge of individual laser control in a solid. Thus, BSM-SG combines elements from both worlds—static solid-state platforms and ion-based optical control. There’s no indication of patent overlap, as BSM-SG operates in a distinct physical regime.
Spin Defects in Solid State (NV Centers in Diamond)
Another leading approach similar in methodology to BSM-SG is spin centers in solid crystals—the most well-known being the negatively charged nitrogen-vacancy center in diamond (NV center). Companies like Quantum Brilliance develop quantum devices using NV centers, capable of room-temperature operation. Here, the quantum carrier is the electron spin in the defect (NV center has spin S=1).
Control: NV centers are controlled via microwave pulses—under an applied static magnetic field (~a few mT), the ms=0 and ±1 energy levels split, and a microwave signal (~2–3 GHz) can switch between |0⟩ and |1⟩ states (magnetic resonance). A green laser (~532 nm) is used for spin initialization—NV is optically pumped into the ms=0 state.
Readout: NV spin states are read optically—fluorescence is spin-dependent. If the spin is in |1⟩ (ms=±1), the defect often transitions to a dark triplet state and emits fewer photons under green illumination; if in |0⟩ (ms=0), fluorescence is bright. This allows optical readout via photodiodes or photomultipliers. This spin-dependent photoluminescence makes NV qubits operable even at high temperatures.
Architectural features: NV centers are embedded in the diamond lattice—either singly or in arrays controlled via implantation. These systems do not require vacuum or cryogenic cooling (can operate at 300 K), enabling compact “quantum accelerators” in standard environments. For improved coherence, lower temperatures (~77 K) and isotopically pure $^{12}$C diamonds are sometimes used.
Quantum Brilliance has demonstrated prototypes with several NV qubits integrated with classical controllers (CPU/GPU) in rack systems. Compatibility: NV centers are gate-based qubits and support quantum logic operations, making them compatible with frameworks like Qiskit. Quantum Brilliance offers its own SDK (Qristal) and simulator, with applications compiled into necessary control sequences. Patents: The company patents methods for integrating NV centers, special resonators, readout techniques, and more—e.g., photonic diamond chips.
Comparison with BSM-SG: NV centers are conceptually close to BSM-SG—both use spin, microwave control, and optical readout. The main difference is the specific defect: BSM-SG uses Yb³⁺ in a crystal, while NV is a nitrogen-vacancy in diamond. Yb³⁺ has distinct energy levels and emits in the infrared, whereas NV operates in the visible spectrum. The ODMR (optically detected magnetic resonance) principle is shared. BSM-SG likely draws inspiration from NV technologies but represents a novel implementation with potential advantages (narrower lines, longer spin coherence). There is no apparent patent conflict, as BSM-SG uses different materials and transitions.
Comparison and Conclusion
BSM-SG shares the most traits with platforms that use spin-based quantum carriers and optical detection. It is conceptually close to NV centers and ion-trap quantum computers: all utilize internal spin transitions in ions/defects and photon-based readout. BSM-SG combines these ideas—using a rare-earth ion (Yb³⁺) in a solid matrix, positioning it between gas-phase ion traps and solid-state spin defects. This is a relatively unique niche.
With IonQ/ion traps: The similarity lies in using Yb and optical readout, along with magnetically insensitive “clock” transitions. IonQ uses lasers for gates, while BSM-SG uses microwaves. BSM-SG avoids complex vacuum systems but faces challenges in addressing individual ions. Overall, the approaches seem independently developed and based on distinct physical regimes.
With NV/spin defects: Strongest overlap—both use spin, microwave control, and optical readout. Yb³⁺ in a crystal can be seen as an "artificial atomic defect" similar to NV. BSM-SG likely draws from ODMR and integration ideas developed for NV but implements them with distinct physics (e.g., infrared emission, different energy levels).
With Equal1/silicon spins: Both use individual spins in solids and aim for monolithic integration with classical electronics. The difference is in signal type—BSM-SG uses optical, Equal1 uses electrical readout. They originate from different scientific domains (rare-earth spectroscopy vs. CMOS quantum electronics), and complement each other as alternative quantum hardware pathways.
With superconducting platforms (IBM, Ocelot, D-Wave): Least overlap—BSM-SG does not use superconducting circuits or currents but atomic transitions. The only commonality is the use of microwave control, though the physics differs (magnetic resonance vs. Josephson junctions). Ocelot’s “cat state” architecture and IBM/Google’s transmons are conceptually unrelated to BSM-SG.
Conclusion: BSM-SG sits at a crossroads of several quantum technologies. It draws on ideas from ion traps and spin defects (atomic transitions and optical methods) but realizes them in a unique way (solid-state matrix with Yb³⁺ and microwave gates). Its similarities to other platforms stem from applying universal quantum principles, not from copying. There is no public evidence of patent disputes—BSM-SG appears to be an independently developed approach inspired by scientific literature, combining components in an original manner. If successful, it could fill a niche between superconducting and ion-optical quantum computers, offering a compact solution with minimal cryogenics and built-in optoelectronics.
2025 Victor Pronchev